Hydrogen Inhalation Therapy And Its Role In Lung Cancer
November 18, 2022 2022-11-28 7:27Hydrogen Inhalation Therapy And Its Role In Lung Cancer
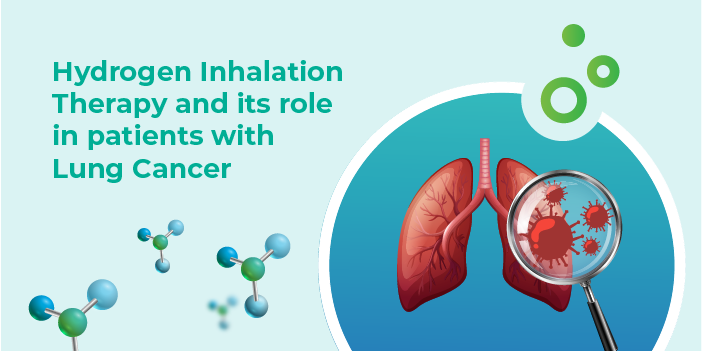
Hydrogen Inhalation Therapy And Its Role In Lung Cancer
Table of Contents
Hydrogen Inhalation Therapy And Its Role In Lung Cancer
Introduction
Oxygen, carbon monoxide, nitric oxide, hydrogen sulfide, and other gases make up gas signaling molecules (GSMs), which are essential for controlling signaling and maintaining cellular homeostasis. Interestingly, these compounds demonstrated the ability to treat cancer through a variety of administration routes. Hydrogen gas (molecular formula: H2) has recently come to light as yet another GSM with a range of biological properties, including anti-inflammatory, anti-ROS, and anti-cancer actions. Growing evidence indicates hydrogen’s potential for extensive and efficient application in clinical therapy, including the reduction of side effects associated with conventional chemotherapeutic medications as well as the inhibition of the growth of cancer cells and xenograft tumors (Li et al., 2019).
Hydrogen as a novel therapeutic agent
Every year, thousands of people would come to drink “holy water” from an abandoned mining pit in Germany, hoping to cure their illnesses. A group of kids suffering from leukemia was brought in to drink the holy water in 1986. After a few months, their symptoms started to significantly improve, and completed recovery was observed in one case. The high hydrogen content of the holy water was thought to be the reason for these improvements. However, survey data from 82 Chinese cancer patients published in 2019, presented the first evidence for “hydrogen oncology” (Chen et al., 2020).
A number of studies have established the efficacy of hydrogen as a therapeutic agent. An experiment on mice with skin tumors, who inhaled a mixture of hydrogen (97.5%) and oxygen (2.5%), showed marked regression in the tumors (Dole et al., 1975). Hydrogen has been tested both in vivo and in vitro to prove its use as a safe and effective antioxidant (Ohsawa et al., 2007). Type 2 diabetes mellitus patients also benefited from drinking hydrogen water (Kajiyama et al., 2008). Hydrogen water supplementation decreases cholesterol and may have a preventive role in metabolic syndrome (Song et al., 2013). Hydrogen water improved UPDRS scores of patients with Parkinsonism in a study in Japan (Huang et al., 2018). An energy metabolic pathway flip from oxidative phosphorylation to aerobic glycolysis is linked to allergic airway inflammation. By flipping this switch back, hydrogen reduces inflammation of the airways in asthma and allergic airway inflammation (Niu et al., 2020) Hydrogen may also have implications in the treatment of gastric cancers (Zhu et al., 2021).
Figure 1
Hydrogen therapy is a promising potential therapeutic option for a wide range of diseases (Figure 1). Gas inhalation as a disease therapy has recently gained popularity, and the list of therapeutic gases continues to expand. For unmet medical needs that currently place a heavy burden on people’s health, hydrogen has the potential to make a big difference as a unique and revolutionary treatment tool (Huang et al., 2010).
Mechanism of Action of Hydrogen
Eliminates reactive oxygen species (ROS)
Several key factors in cancer, including ROS and antioxidant enzymes, are regulated by hydrogen. ROS are a group of oxygen-containing molecules that can harm DNA/RNA and proteins resulting in severe damage and apoptosis. External chemical attacks or an imbalance of regulatory systems result in excessive ROS production. During cancer, H2 inhalation selectively scavenges most cytotoxic ROS, such as hydroxyl radicals (OH) and proximities (ONOO), which play a causal role in tumor cell proliferation, invasion, and metastasis. By increasing the expression of antioxidant enzymes (SOD, HO-1, and Nrf2), hydrogen treatment enhances the elimination of ROS (Li et al., 2019)
Modulates Inflammation
Chemotherapy-induced inflammation in cancer patients leads to cancer spread and treatment failure in addition to causing serious adverse effects. Hydrogen can stop the growth and spread of tumors and lessen the negative effects of chemotherapy and radiotherapy by controlling inflammation. Tumor necrosis factors (TNFs), which are released by macrophages, and interleukins (ILs), which are released by leukocytes, are examples of typical inflammatory cytokines. Both have been linked to the development & spread of cancer, and hydrogen gas can block both ILs (especially IL-8, IL-1β) and TNFs (especially TNF-α) (Li et al., 2019).
Enhances/Restores Mitochondrial Function
During cancer, the mitochondria of T cells are destroyed. This loss of activity is due to the Inactivation of mitochondrial peroxisome proliferator-activated receptor γ coactivator 1α (PGC‑1α) (Kamphorst et al., 2017). H2 stimulates the gene expression of (PGC-1α) (Kamimura et al., 2016). Hydrogen can permeate into lymphocyte mitochondria as an antioxidant gas and selectively scavenge oxygen free radicals (Ohta, 2012). In 2019, Akagi and Baba observed an increase in T cells of cancer patients due to hydrogen inhalation. They further investigated whether the rise in T cell level was due to mitochondrial restoration. They were able to prove an increase in mitochondria of stage four lung cancer patients by using CoQ10 as a marker for mitochondrial restoration (Akagi and Baba, 2022).
Boosts Immune System
Aging of the immune system, chemotherapy itself, and other tumor-related factors are the main reasons for cancer recurrence and metastasis. Immune reconstitution with immune-enhancing drugs is carried out traditionally to avoid relapse after standard treatment. Hydrogen inhalation can be used for immune reconstitution. After 2 weeks of inhalation of a mixture of hydrogen and oxygen (66.7%: 33.3%) four hours per day; functional, cytotoxic, and helper T cells, NKT cells, Th1, natural killer cells, and Vδ2 cells increased in number while exhausted and senescent cytotoxic T cells decreased and restored back to the normal level (Chen et al., 2020).
Genes and Signaling Pathways associated with Lung cancer
Lung cancer accounts for 18% of all cancer-related deaths in the world (Sung et al., 2021). About 85% of lung cancers are non-small cell lung cancers (NSCLC) (Nawaz and Webster, 2016). Tobacco smoke is the cause of 85% of lung cancers. The smoke results in invasive and pre-invasive lesions, furthermore metastasis due to the gradual accumulation of irregularities in the genetic and epigenetic system. About one-fifth of the adenocarcinomas are not related to tobacco smoke at all. (Sun et al., 2007).
The carcinogens in tobacco smoke are significantly linked to the development of lung cancer and cause genetic changes by forming DNA adducts (Hecht, 1999). However, less than 20% of smokers develop lung cancer, suggesting a genetic predisposition to the disease (Sun et al., 2007).
Immunotherapy for Lung Cancer
In cases of advanced NSCLC, when patients no longer respond to targeted therapy, Immunotherapy or a combination of chemotherapy with immunotherapy is employed (Lung cancer – Non-Small Cell- Types of Treatment, 2022).
PD-1 Pathway Inhibitors
Many cancers overexpress programmed cell death-ligand (PD-L1). The expression of Certain ligands on the surface of tumor cells helps them dodge the T cells by their action on particular immune checkpoint pathways. One such pathway is programmed cell death (PD-1). PD-1 acts as an immune checkpoint receptor on the membrane of T cells. (El-Guindy et al., 2018). Inhibition of these checkpoints (PD-1/PD-L1) with immune checkpoint inhibitors (ICIs) prevents the interaction with the receptor on T cells and the ligand on cancerous cells (Rittmeyer et al., 2017) Herbst, 2016). This inhibition has improved the prognosis of many cancers that are extremely difficult to treat. It has provided dramatic results in the treatment of small cell lung cancer (SCLC) and non-small-cell lung cancer (NSCLC) (Paz-Ares et al., 2019).
Certain tumors can be effectively treated by therapies inhibiting PD-1 that leads to an anticancer immune response by rejuvenating T cells as PD-1 is believed to suppress signal transduction via the T cell receptor (TCR). Hui et al. discovered that PD-1 acts through CD28 instead of TCR. Kamphorst et al. also discovered the same during their experiments on viral infections in mice. Lung cancer patients who received PD-1 therapy also had an increased number of CD8 T cells. CD 28/B7 pathway plays an important role in CD8 T cell proliferation while treating cancer patients with PD-1 therapy ((Kamphorst et al., 2017).
Hydrogen also helps in the restoration of CD8+ T cells by stimulation of mitochondrial PGC-1α. Nivolumab belongs to PD-1 inhibitors and exerts synergistic effects with mitochondrial stimulants. Hydrogen was studied for its synergistic effects on the activity of nivolumab in lung cancer patients. 56 patients receiving Nivolumab were included in the study, out of which 42 patients received hydrogen gas in combination with the drug. The overall survival (OS) of patients receiving combination therapy was significantly longer as compared to the control group. Exhausted CD8+ T cells lead to a poor prognosis in OS. Improvement in mitochondrial function, marked by higher CoQ10, could be linked with improvement in OS. The clinical efficacy of nivolumab is enhanced by the improvement of the mitochondrial function of CD8+ T cells (Akagi and Baba, 2022).
CD47-SIRPα pathway Inhibitors
Another important immune checkpoint for lung cancer is the CD47-SIRPα axis. Zhao et al. discovered the overexpression of CD-47 in NSCLC tumor tissues. Potent antitumor activity of SIRPα–Fc mAb against NSCLC has also been proven by its targeting of CD-47 (Figure 2). It also triggered autophagy via Akt/mTOR pathway inactivation and acted as a cytoprotectant in NSCLC cells. Human trials for the efficacy of CD-47 inhibitors in NSCLC have not been conducted yet (Catalán et al., 2020).
Figure 2
Targeted Therapy for Lung Cancer
Targeted therapy for lung cancer is directed against the specific proteins and gene targets that contribute to cancer growth and survival (Lung cancer – Non-Small Cell- Types of Treatment, 2022).
Tumor Suppressors
PD-L1 expression is reduced by preserved p53 (pro-apoptotic protein) role. Mutations in the gene encoding for p53 result in loss of its function and are linked with higher expression of PD-L1. When mutations in KRAS and TP53 (p53 encoder) occur at the same time in NSCLC, these effects are intensified.
Mutations in Serine/threonine kinase 11 (STK11)- a tumor suppressor- are also found in lung adenocarcinoma, especially when KRAS mutations are also present. PD-L1 expression is low or non-existent in case of mutation or loss of STK11, resulting in impaired efficiency of PD-1/PD-L1 targeting ICIs (Lamberti et al., 2020).
RAS and Mitogen-Activated Protein Kinase
About one-third of lung adenocarcinomas have activating mutations in Kirsten Ras Sarcoma Viral Oncogene Homolog (KRAS). RAS activation stabilizes mRNA of PD-L1 and inhibits tristetraprolin, resulting in increased expression of PD-L1 in cell lines of lung cancer.
PI3K/Akt/mTOR
PI3K/Akt/mTOR pathway is distorted in NSCLC as well as numerous types of cancers. Under normal conditions, this pathway causes autophagy of damaged self-cells. Activated mTOR leads to increased PD-L1 protein.
Receptor Tyrosine Kinases
The expression of PD-L1 on NSCLC cells is induced by ligand binding and subsequent activation of the epidermal growth factor receptor (EGRF). Lung adenocarcinomas have mutated EGRF in 10-20% of cases. This mutation is usually present in nonsmokers, females and Asians. Mutated EGFR can intensify the expression of PD-L1. EGFR inhibitors are included in targeted therapy for lung cancer (Lamberti et al., 2020).
Upregulation of CD47 expression was also observed in NSCLC cell lines with EGFR mutations (Catalán et al., 2020)
Genes and pathways targeted by Hydrogen?
H2 directly scavenges hydroxyl radicals (OH). H2 also has antioxidant, anti-inflammatory, and apoptotic effects by indirectly regulating gene expression. H2 may have antitumor properties through these direct and indirect actions (Figure 3)
Figure 3
Wang et al. carried out in vivo and in vitro experiments to study the inhibitory effect and mechanism of the action of hydrogen gas on lung cancer. He used human cancer cell lines (A549 and H1975) for in vivo studies and used A549 xenografted mice for in vitro studies. Increasing the concentration of hydrogen from 20% to 80% resulted in a subsequent increase in apoptosis and a corresponding inhibition of proliferation in vitro and significantly arrested tumor growth in vivo. The expression of tumor necrosis factor-α (TNF-α), SOD, IL-1β, IL-8, and IL-13 in lung tissue was amplified due to hydrogen gas, and the expression of ROS was suppressed. Hydrogen gas also confirmed SMC3 repression upon immunohistochemical staining of samples from the mice. The SMC3 gene is a regulator of chromosome condensation. This study concluded that the downregulation of SMC3 could be the underlying mechanism for the antitumor effect of hydrogen (Wang et al., 2018).
Hydrogen may inactivate extracellular signal-regulated kinase (ERK) which is necessary for the transcription of the vascular endothelial growth factor (VEGF) gene. VEGF plays a key role in tumor angiogenesis. Low levels of VEGF due to electrolyte-reduced water inhibited angiogenesis (Ye et al., 2008).
The combination of 5-fluorouracil with hydrogen water improved survival rates in colorectal cancer. This combination increased the expression of phosphorylated adenosine monophosphate-activated protein kinase (p-AMPK), caspase-3, and apoptosis-inducing factor (AIF) than using either of them alone. Antitumor activity of hydrogen water was attributed to its role in the activation of the apoptotic pathway (Runtuwene et al., 2015).
In endometrial cancer, hydrogen protects normal cells while destroying cancerous cells via the pyrotrophic pathway. This pathway acts via the formation of Terminal protein gasderminn D (GSDMD) (Yang et al., 2020).
Results of in-vitro studies on lung cancer suggested the involvement of CD47 in the suppression of lung cancer due to hydrogen. The effect of CD47 on lung cancer suppression due to hydrogen gas was further examined. A direct link between the dose of hydrogen gas and the reduction in CD47 and CDC42 expression was determined. H2 could not produce an antitumor effect when the cancerous cell lines upregulated CD47. However, marked improvement in tumor resolution occurred upon downregulation of CD47. H2 treatment in vivo suppressed CDC42 and CD47 expression and enhanced phagocytosis in-vitro (Meng, 2020).
Hydrogen Inhalation in Lung cancer with Brain Metastasis
Although brain metastases are a frequent consequence of a variety of malignancies, patients with lung cancer are more likely to experience them than other cancer patients. Brain metastases are present in about 10% of individuals with newly diagnosed NSCLC. Lung cancer is the main tumor in between 40 and 50 percent of patients with brain metastases. Treatment choices for patients with brain metastases are changing for a variety of reasons, even though there are well-established management strategies for these conditions. First, many patients with advanced NSCLC live for several years, giving brain metastases and side effects from earlier treatments for brain metastases more time to develop. Second, patients with more prevalent driver mutations, notably activating mutations of the EGFR and anaplastic lymphoma kinase (ALK) rearrangements, are more likely to develop brain metastases. Third, more recent targeted treatments for EGFR and ALK have shown significantly higher intracranial efficacy, allowing for cautious consideration of systemic therapy as a preferred front-line strategy over previously recommended local treatment. (Yuan et al., 2022).
Hydrogen gas is an excellent candidate to be used in NSCLC that’s metastasized to the brain because it can easily cross the blood-brain barrier which is a limitation of standard chemotherapy. Hydrogen also shows neuroprotective effects by diminishing COX2 activity (Hou et al., 2012) or by antiapoptotic protein kinase activation (Yang et al., 2018). Inhibition of JNK and caspase-3 (proapoptotic factors) is caused by H2 (Sun et al., 2011) It downregulates many proinflammatory cytokines as well (Zhang et al., 2012).
One case study on a 44-year-old female presented evidence for the use of hydrogen monotherapy in controlling tumors. The patient initially presented with lung cancer with multiple metastases in November 2015. She received oral targeted drugs after brain metastases removal. Most of the lesions remained stable for more than 2 years. Her condition started to get worse in March 2018. Cancer had metastasized to multiple organs including the brain, adrenal gland, liver, and bones. There was an accumulation of hydrocephalus in the third and lateral ventricles (Figure 4A). The patient refused radiotherapy and brain surgery at this point. She signed informed consent for hydrogen inhalation therapy alone. She inhaled a mixture of hydrogen (67%) and oxygen (33%) using an AMS-H-03 hydrogen oxygen nebulizer from China. She experienced relief in her symptoms during the first month of hydrogen therapy. Hydrocephalus accumulation significantly reduced in the third and lateral ventricles and the brain tumors showed a significant reduction in size after four months of hydrogen monotherapy (Figure 4B). One year into the therapy, all brain tumors disappeared (Figure 4C). Liver and lung metastases showed no significant change. This data proved that hydrogen therapy helped in prolonging the survival time after the failure of standard treatments (Chen et al., 2019).
The possible mechanism of action of hydrogen for the control of brain metastasis in NSCLC was through MAPK, TCF/LEF-1, DVL-1/3 of Wnt/β-catenin and SIRT1 (Chen et al., 2019).
Figure 4
In-vivo and In-vitro studies/ Human Trials/ Case Studies
A survey reported the effect of hydrogen inhalation in 82 cancer patients with stage 3 and stage 4 cancer. These patients inhaled hydrogen from AMS-H0-03 Hydrogen-oxygen Atomizer at their homes or at the hospital. Improvement in quality of life was observed after one month of hydrogen inhalation. Pain, insomnia, fatigue, and appetite improved in patients inhaling the Hydrogen-oxygen mixture. The performance status of Lung cancer patients had the highest improvement rate. Tumor markers also decreased with Hydrogen therapy, with Lung cancer having the highest rate of tumor marker decrease. The disease control rate was 47.7% for stage 4 cancer patients and it was much higher (83%) for stage 3 cancer patients (Chen et al., 2020)
Chen et al. conducted the first clinical trial to prove the efficacy of hydrogen therapy in patients with advanced NSCLC. Patients were divided into 5 treatment groups (control group, H2 only, Immuno-H2, Target-H2, Chemo-H2). Patients in the control group inhaled only O2 while patients in the remaining four groups inhaled a mixture of 66.7% hydrogen with 33.3% oxygen (4-5h/day) for 5 months or till cancer recurrence. Before starting the trial, the pulmonary symptoms of all the groups were not significantly different. During the study period, the frequency of symptoms of all groups other than the control group decreased gradually. The group that received H2 therapy alone, showed significant improvement in tumor-related symptoms, as shown in figure 5.
Figure 5
The groups that were receiving drug therapy in combination with hydrogen therapy showed similarity in the tumor-related symptoms but drug-related symptoms were not similar at the beginning of this trial. All drug-related symptoms improved significantly over the course of treatment except diarrhea, as shown in figure 6.
Figure 6
Febrile granulocytopenia, insomnia, oral mucositis, maculopapular rash, and nausea and vomiting decreased significantly in the target-H2 therapy group. Anorexia, febrile granulocytopenia, anemia, diarrhea and constipation, and thrombocytopenia decreased significantly in the Chemo-H2 therapy group.
While patients in all treatment groups developed tumor progression, the progression-free survival (PFS) of patients receiving hydrogen therapy combined with the traditional therapies was significantly longer compared to the control group. The longest PFS was observed in the group receiving a combination of immunotherapy and hydrogen. This study proved the efficacy of hydrogen in reducing the adverse effects of cancer medication and provided clinical evidence for use of hydrogen inhalation to control tumor progression in lung cancer (Chen et al., 2020).
Conclusion
Hydrogen exerts synergistic effects with PD-1 inhibitors in lung cancer patients. It reduces the side effects of chemotherapy, immunotherapy, and targeted therapy in NSCLC patients and may also act as an innate immune checkpoint inhibitor through its action on CD47. Hydrogen monotherapy can be beneficial for advanced NSCLC patients in which all other therapies have failed and may prolong survival. Downregulation of SMC3 expression could be the reason for the antitumor activity of hydrogen.